Understanding Cooling Load Computation: A Comprehensive Guide for Architecture Students -Architectural Cooling Load Calculation
- Architect Dennis
- Nov 29, 2024
- 6 min read
Updated: Dec 4, 2024
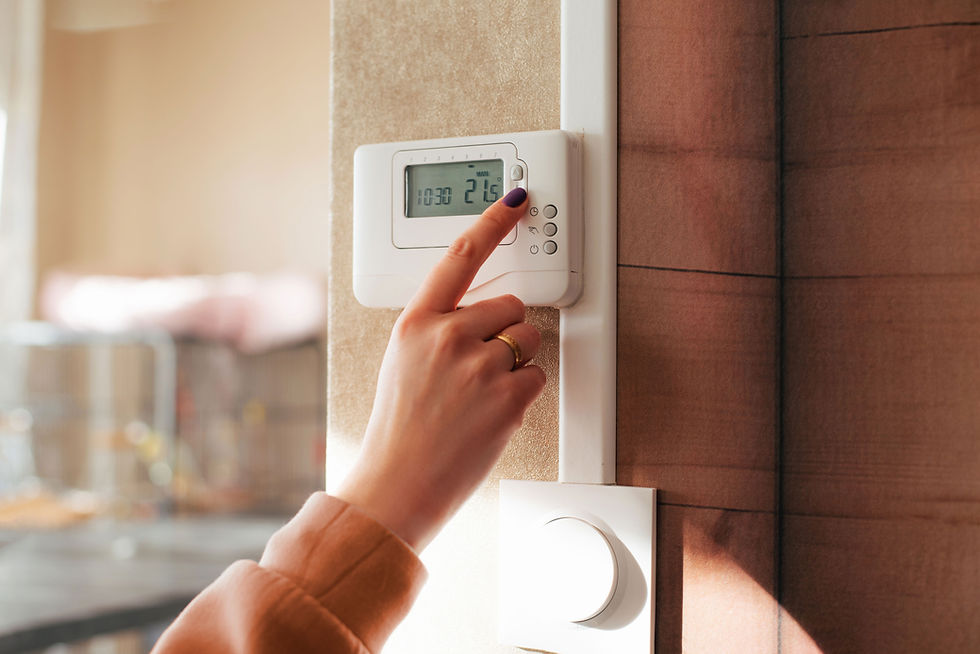
Cooling load computation is a critical skill for architects and building designers, representing the amount of heat that must be removed from a space to maintain a comfortable indoor temperature. This calculation is fundamental to designing energy-efficient and thermally comfortable buildings.
What is Cooling Load?
Cooling load is the rate of heat transfer that must be eliminated from a space to maintain a desired indoor temperature and humidity level. It encompasses various heat sources that contribute to warming a building, including:
Solar radiation
Internal heat generation from occupants
Heat from electrical equipment
Walls, roof, and window heat transmission
Ventilation and infiltration heat gain
Key Components of Cooling Load Calculation -Architectural Cooling Load Calculation

Heat Transmission through Building Envelope
Wall Heat Transmission
Heat transmission through walls depends on:
Wall material properties
Thickness of the wall
Temperature difference between indoor and outdoor environments
Solar radiation absorption
Calculation Formula:
Q(wall) = U x A x ΔT
Where:
Q(wall) = Heat transmission through wall (Watts)
U = Overall heat transfer coefficient (W/m²K)
A = Wall area (m²)
ΔT = Temperature difference (°C)
Refer to the table below for Overall heat transfer coefficient (W/m²K)
Wall Type | Construction Details | U-value (W/m²K) | Typical Applications |
Single Brick Wall | 230mm solid brick | 2.0 | Older buildings, unrenovated structures |
Cavity Brick Wall | Unfilled cavity, 102mm outer leaf, 102mm inner leaf | 1.6 | Traditional construction |
Insulated Cavity Wall | Cavity filled with mineral wool insulation | 0.55 | Modern residential construction |
Concrete Block Wall | 200mm dense concrete block, uninsulated | 2.5 | Industrial and older commercial buildings |
Insulated Concrete Block Wall | 200mm block with 50mm internal insulation | 0.6 | Modern energy-efficient buildings |
Timber Frame Wall | 140mm timber frame with mineral wool insulation | 0.3 | Eco-friendly and passive house designs |
Structural Insulated Panel (SIP) | Insulation core with structural facing | 0.2 | High-performance energy-efficient buildings |
Double Brick Wall | 230mm solid brick with air gap | 1.4 | Historical buildings with some thermal performance |
Lightweight Concrete Wall | 150mm aerated concrete block | 0.7 | Modern lightweight construction |
Glass Reinforced Concrete (GRC) | 100mm thick with minimal insulation | 1.8 | Contemporary architectural applications |
Typical Temperature Differences (ΔT)
Mild Climate: 10-15°C
Hot Climate: 15-25°C
Extreme Climate: 25-35°C
Factors Affecting U-value
Wall material composition
Thickness of materials
Presence and type of insulation
Air gaps and cavities
Surface conditions
Material thermal conductivity
Recommendations for Architects
Always use the most recent and locally relevant U-value data
Consider local building codes and energy efficiency standards
Consult with thermal performance specialists
Use computational tools for precise calculations
Account for material aging and performance degradation
Note: U-values can vary slightly based on specific material brands, manufacturing processes, and local standards. Always verify with manufacturer specifications and local building regulations.
Solar Heat Gain through Windows
Windows are significant contributors to cooling load due to their high solar heat transmission to compute for the Architectural Cooling Load Calculation.
Calculation Formula:
Q(window) = SHGC x A x I x SC
Where:
Q(window) = Solar heat gain (Watts)
SHGC = Solar Heat Gain Coefficient
A = Window area (m²)
I = Solar radiation intensity (W/m²)
SC = Shading coefficient
Refer to the table below for Solar Heat Gain Coefficient (SHGC) by Window Type
Window Type | SHGC Range | Typical Applications | Performance Characteristics |
Single Clear Glass | 0.80 - 0.90 | Older buildings | High heat transmission |
Double Clear Glass | 0.65 - 0.75 | Standard residential windows | Moderate heat control |
Low-E Double Glazing | 0.40 - 0.55 | Energy-efficient buildings | Reduced solar heat gain |
Low-E Triple Glazing | 0.30 - 0.45 | Passive house standards | Minimal heat transmission |
Reflective Coated Glass | 0.20 - 0.35 | Commercial buildings | Significant heat rejection |
Spectrally Selective Glass | 0.25 - 0.40 | High-performance applications | Advanced thermal control |
Refer to the table below for Solar Radiation Intensity (I) by Geographic Regions and Seasons
Region/Climate | Summer Intensity (W/m²) | Winter Intensity (W/m²) | Peak Hours | Notes |
Tropical Regions | 800 - 1,000 | 500 - 700 | 10 AM - 2 PM | Consistent high radiation |
Desert Regions | 900 - 1,100 | 600 - 800 | 11 AM - 3 PM | Extreme solar exposure |
Temperate Zones | 600 - 800 | 300 - 500 | 11 AM - 1 PM | Moderate seasonal variation |
Mediterranean | 750 - 950 | 450 - 650 | 10 AM - 2 PM | Mild climate conditions |
Northern Regions | 500 - 700 | 200 - 400 | 12 PM - 2 PM | Limited solar intensity |
Refer to the table below for Shading Coefficient (SC) by Shading Strategy
Shading Type | SC Value | Reduction Effectiveness | Typical Applications |
No Shading | 1.0 | 0% | Unprotected windows |
Light Exterior Curtains | 0.7 - 0.8 | 20-30% | Residential spaces |
Exterior Horizontal Louvers | 0.5 - 0.6 | 40-50% | Modern architectural design |
Deep Overhangs | 0.4 - 0.5 | 50-60% | Passive solar design |
External Roller Screens | 0.3 - 0.4 | 60-70% | High-performance buildings |
Vegetation Shading | 0.4 - 0.5 | 50-60% | Sustainable design approaches |
Internal Heat Gains
Occupant Heat Generation
Average human generates approximately 100W of heat
Varies based on activity level
Equipment Heat Generation
Equipment and Lighting Heat Generation Reference Table
Refer to the table below for Lighting Heat Generation by Type
Lighting Type | Typical Wattage | Heat Generation (W) | Efficiency | Typical Application |
Incandescent Bulb | 60W | 55-58 | Low | Residential, older fixtures |
Halogen Bulb | 50W | 45-50 | Moderate | Task lighting, accent lighting |
Compact Fluorescent (CFL) | 15W | 10-12 | Moderate | Energy-efficient residential |
LED Bulb | 9W | 3-5 | High | Modern, energy-efficient spaces |
Fluorescent Tube (4ft) | 32W | 25-30 | Moderate | Office, commercial spaces |
Metal Halide Lamp | 400W | 350-380 | Low | Industrial, large spaces |
High-Pressure Sodium Lamp | 250W | 220-240 | Low | Street lighting, warehouses |
Refer to the table below for Office and Commercial Equipment Heat Generation (Computing Devices)
Device | Average Heat Generation (W) | Usage Scenario |
Desktop Computer | 100-250 | Standard office work |
Laptop | 50-100 | Mobile/flexible workspaces |
Tablet | 10-20 | Light computing tasks |
Server (Rack Unit) | 300-500 | Data centers |
Refer to the table below for Office and Commercial Equipment Heat Generation (Office Electronics)
Device | Average Heat Generation (W) | Usage Scenario |
Printer (Laser) | 200-300 | High-volume printing |
Multifunction Printer | 150-250 | Office environments |
Monitor (LCD 24") | 30-50 | Standard office display |
Projector | 200-300 | Presentation rooms |
Refer to the table below for Residential Appliances Heat Generation (Kitchen Appliances)
Appliance | Average Heat Generation (W) | Operation Time |
Refrigerator | 100-150 | Continuous |
Microwave Oven | 600-1200 | Short duration |
Electric Stove (Single Burner) | 1000-1500 | Intermittent |
Dishwasher | 300-500 | 1-2 hours |
Refer to the table below for Residential Appliances Heat Generation (Laundry and Cleaning)
Appliance | Average Heat Generation (W) | Operation Time |
Washing Machine | 350-500 | 30-60 minutes |
Clothes Dryer | 2000-5000 | 45-90 minutes |
Vacuum Cleaner | 200-300 | Short duration |
Refer to the table below for Special Purpose Spaces (Laboratory/Technical Spaces)
Equipment | Heat Generation (W) | Usage Context |
Scientific Instrument | 200-1000 | Research facilities |
3D Printer | 100-300 | Design studios |
Large Computer Workstation | 250-500 | Graphic design |
Key Considerations for Architects
Actual heat generation varies with: Device age, Usage intensity, Ambient temperature Actual heat generation varies with:
Device age
Usage intensity
Ambient temperature
Modern devices tend to be more energy-efficient
Consider diversity factors in large spaces
Account for future technology changes
Note: Values are representative averages. Precise measurements should be taken for specific equipment and usage scenarios.
Sample Cooling Load Computation Problem
Problem Scenario
Design a cooling system for a classroom:
Room dimensions: 8m x 6m x 3m
Window area: 4m²
Orientation: South-facing
Occupancy: 25 students
Location: Moderate climate zone
Mild Climate: 10-15°C
Step-by-Step Calculation
Wall Heat Transmission
Insulated Concrete Block Wall
Wall U-value: 0.5 W/m²K
Wall area: (2 x 8m + 2 x 6m) * 3m = 84 m²
Temperature difference: 15-10= 5°C
Q(wall) = 0.5 x 84 x 5 = 210 W
Window Heat Transmission
Window SHGC (Low-E Double Glazing): 0.4
Window area: 4 m²
Solar radiation (Northern Regions): 500 W/m²
Shading coefficient (Light Exterior Curtains): 0.7
Q(window) = 0.4 x 4 x 500 x 0.7 = 560 W
Occupant Heat Gain
25 users * 100W = 2,500 W
Total Cooling Load
Sum of heat transmission and internal gains
Total = 210W+ 560W+ 2,500W = 3,270 W (or 3.27 kW)
Best Practices
Use accurate material properties
Consider local climate conditions
Account for building orientation
Implement proper shading strategies
Regular model calibration and verification
Cooling load computation is a complex but essential skill for architects.
Sources and References for Cooling Load Computation Data
Comprehensive Reference List
Wall Heat Transfer Coefficient (U-value)
ASHRAE Handbook of Fundamentals (2017)
Building Research Establishment (BRE) Publications
Authoritative source for UK building thermal performance standards
International Energy Agency (IEA) Energy Efficiency Guidelines
Global standards for thermal performance
Solar Heat Gain Coefficient (SHGC) and Radiation Intensity
Lawrence Berkeley National Laboratory - Windows Research
National Renewable Energy Laboratory (NREL)
Comprehensive solar radiation and building energy data
American Society of Heating, Refrigerating and Air-Conditioning Engineers (ASHRAE)
Solar Heat Gain Coefficient Database
Equipment and Lighting Heat Generation
Energy Information Administration (EIA)
Comprehensive energy consumption and equipment data
URL: https://www.eia.gov/
Lawrence Berkeley National Laboratory - End-Use Energy Consumption Research
Detailed studies on equipment energy consumption
URL: https://eta.lbl.gov/
International Energy Agency (IEA) Energy Efficiency Databases
Global equipment energy performance standards
Additional Academic and Professional References
Disclaimer and Methodology
Data Compilation Process
Cross-referenced multiple authoritative sources
Averaged values from different research publications
Considered variations in global building standards
Updated with most recent available research (as of 2024)
Conclusion
Cooling load computation is a complex but essential skill for architects. By understanding the principles and methodically breaking down heat sources, designers can create more energy-efficient and comfortable spaces.
Learn more related topics from our well curated reference from Amazon. Head over to our Resource Page
Comments